La Agencia Espacial Europea (ESA) ha apostado por Valencia para la instalación de un laboratorio pionero y de referencia internacional en el ámbito de las telecomunicaciones espaciales. Se instalará en la Ciudad Politécnica de la Innovación, parque científico de la Universidad Politécnica de Valencia, y en el campus de Burjassot de la Universitat de València-Estudi General.
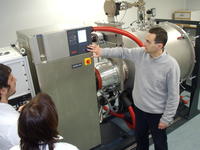
Investigadores del ITEAM de la UPV en el actual laboratorio experimental del Instituto para medidas de alta potencia en comunicaciones espaciales
Este laboratorio, que hasta ahora tenía su sede en Noordwijk (Holanda), se centrará en el estudio de los fenómenos de ruptura de radiofrecuencia en satélites y naves espaciales con señales de microondas de alta potencia así como en la validación de componentes de microondas para aplicaciones espaciales.
Otra de las actividades del laboratorio se enfocará en su apoyo a la industria europea ofreciendo sus recursos e instalaciones para calificar componentes europeos de alta potencia embarcados en satélites y así garantizar las comunicaciones con Tierra.
Val Space Consortium
A finales de 2009, la ESA firmó un preacuerdo con la Generalitat Valenciana –a través de la Conselleria d´Educació-, el Ayuntamiento de Valencia, la Universidad Politécnica de Valencia y la Universitat de València-Estudi General -que formarán el Val Space Consortium- en el que se sentaron las bases para la instalación en nuestra ciudad, durante los próximos meses, del mencionado laboratorio.
El laboratorio experimental se ubicará en las instalaciones de la Universidad Politécnica de Valencia, concretamente en la Ciudad Politécnica de la Innovación, y en la Facultad de Física de la Universitat de València-Estudi General.
Hasta la fecha, este laboratorio ha permanecido en el Centro Europeo de Investigación y Tecnología Espacial (ESTEC), que se encuentra en Noordwijk (Holanda), y que se encarga del diseño de la mayor parte de los satélites y del desarrollo tecnológico de la Agencia Espacial Europea.
Este laboratorio podría convertirse en un primer embrión para el desarrollo de un centro espacial en Valencia que albergaría otras disciplinas directamente relacionadas con la investigación espacial.
El laboratorio de la ESA se convertirá, además, en un foco de atracción de científicos europeos que acudirán a Valencia a formarse y a desarrollar sus investigaciones.
ELABORADO POR:
NERWIN ANTONIO MORA REINOSO
C.I: 17.557.095
CAF